Created by CK-12 Foundation/Adapted by Christine Miller
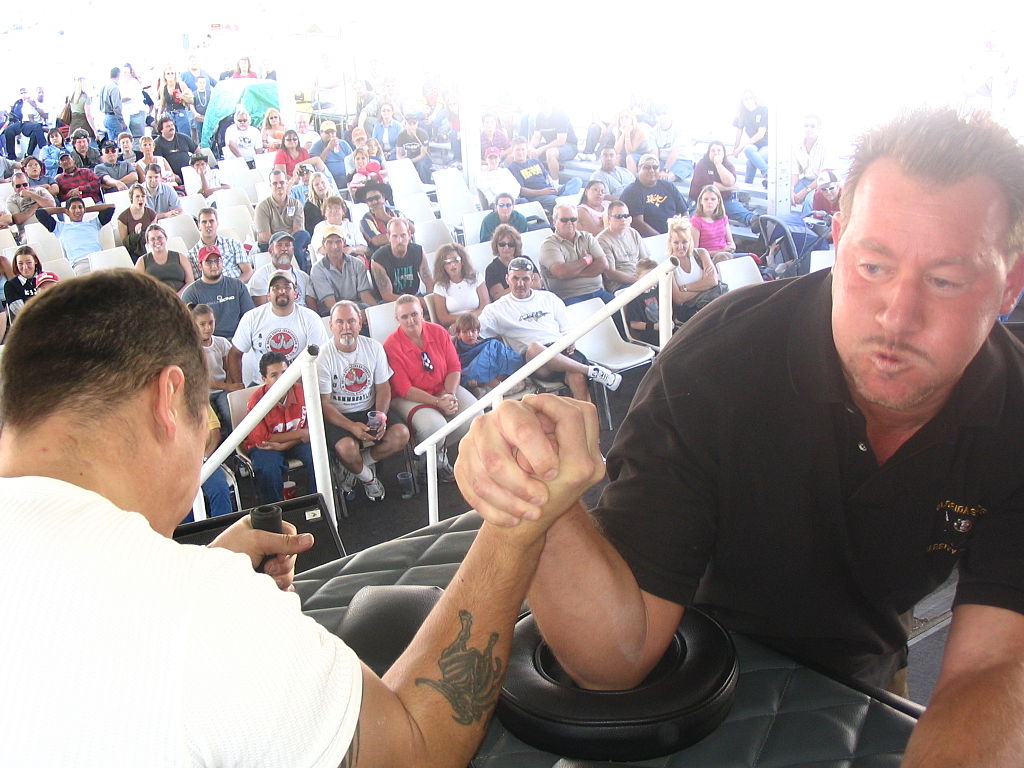
Arm Wrestling
It’s obvious that a sport like arm wrestling (Figure 12.4.1) depends on muscle contractions. Arm wrestlers must contract muscles in their hands and arms, and keep them contracted in order to resist the opposing force exerted by their opponent. The wrestler whose muscles can contract with greater force wins the match.
What Is a Muscle Contraction?
A muscle contraction is an increase in the tension or a decrease in the length of a muscle. Muscle tension is the force exerted by the muscle on a bone or other object. A muscle contraction is isometric if muscle tension changes, but muscle length remains the same. An example of isometric muscle contraction is holding a book in the same position. A muscle contraction is isotonic if muscle length changes, but muscle tension remains the same. An example of isotonic muscle contraction is raising a book by bending the arm at the elbow. The termination of a muscle contraction of either type occurs when the muscle relaxes and returns to its non-contracted tension or length.
To use our arm wrestling example, if both arm wrestlers have equal strength and they are pulling with all their might, but there is no movement, that is isometric muscle contraction. However, as soon as one arm wrestler starts to win and is able to start pulling the opponents arm down, that is isotonic muscle contraction.
How a Skeletal Muscle Contraction Begins
Excluding reflexes, all skeletal muscle contractions occur as a result of conscious effort originating in the brain. The brain sends electrochemical signals through the somatic nervous system to motor neurons that innervate muscle fibres (to review how the brain and neurons function, see the chapter Nervous System). A single motor neuron with multiple axon terminals is able to innervate multiple muscle fibres, thereby causing all of them to contract at the same time. The connection between a motor neuron axon terminal and a muscle fibre occurs at a site called a neuromuscular junction. This is a chemical synapse where a motor neuron transmits a signal to a muscle fibre to initiate a muscle contraction. The process by which a signal is transmitted at a neuromuscular junction is illustrated in Figure 12.4.2 below.
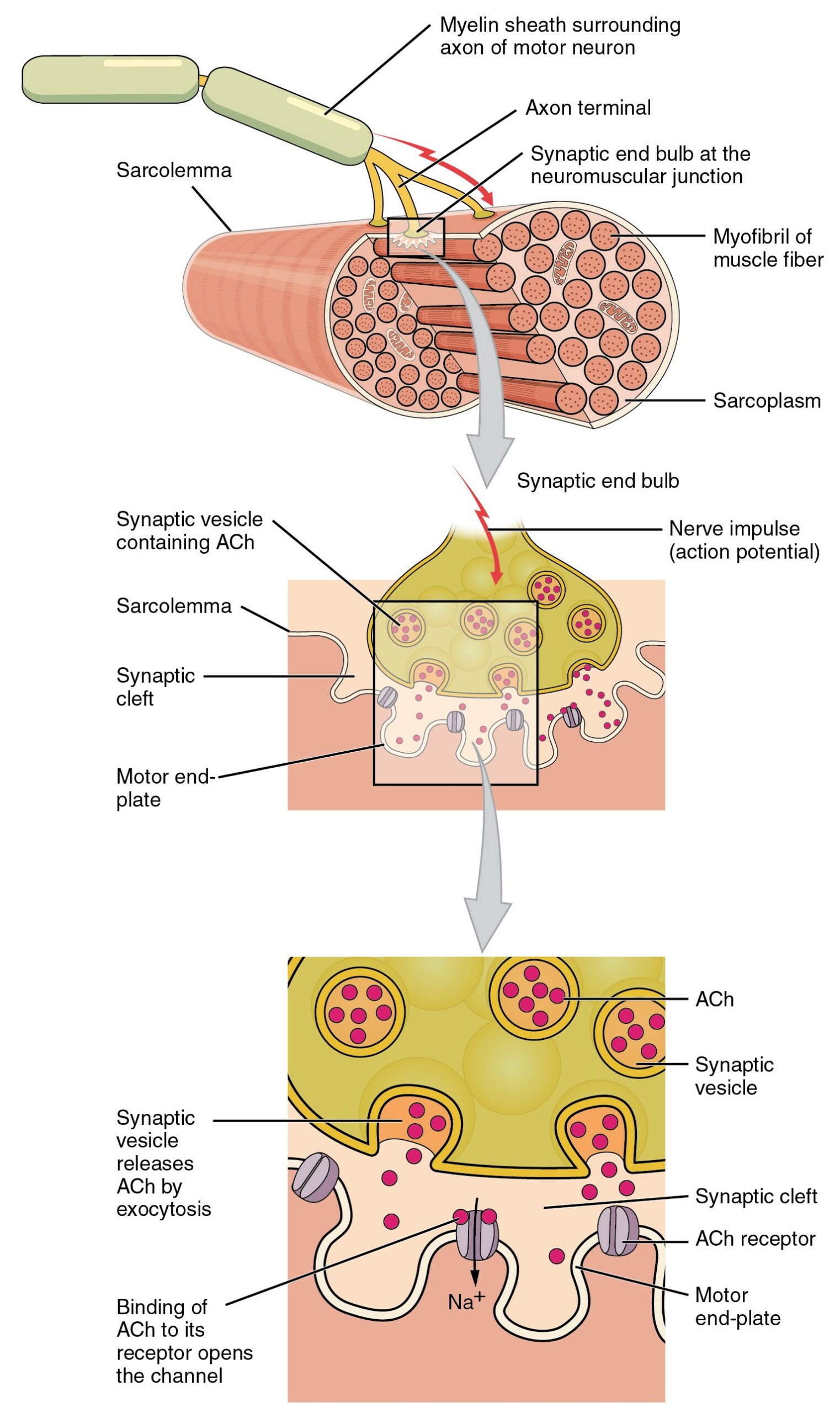
The sequence of events begins when an action potential is initiated in the cell body of a motor neuron, and the action potential is propagated along the neuron’s axon to the neuromuscular junction. Once the action potential reaches the end of the axon terminal, it causes the release of the neurotransmitter acetylcholine (ACh) from synaptic vesicles in the axon terminal. The ACh molecules diffuse across the synaptic cleft and bind to receptors on the muscle fibre, thereby initiating a muscle contraction.
Sliding Filament Theory of Muscle Contraction
Once the muscle fibre is stimulated by the motor neuron, actin and myosin protein filaments within the skeletal muscle fibre slide past each other to produce a contraction. The sliding filament theory is the most widely accepted explanation for how this occurs. According to this theory, muscle contraction is a cycle of molecular events in which thick myosin filaments repeatedly attach to and pull on thin actin filaments, so the filaments slide over one another, as illustrated in Figure 12.4.3. The actin filaments are attached to Z discs, each of which marks the end of a sarcomere. The sliding of the filaments pulls the Z discs of a sarcomere closer together, thus shortening the sarcomere. As this occurs, the muscle contracts.
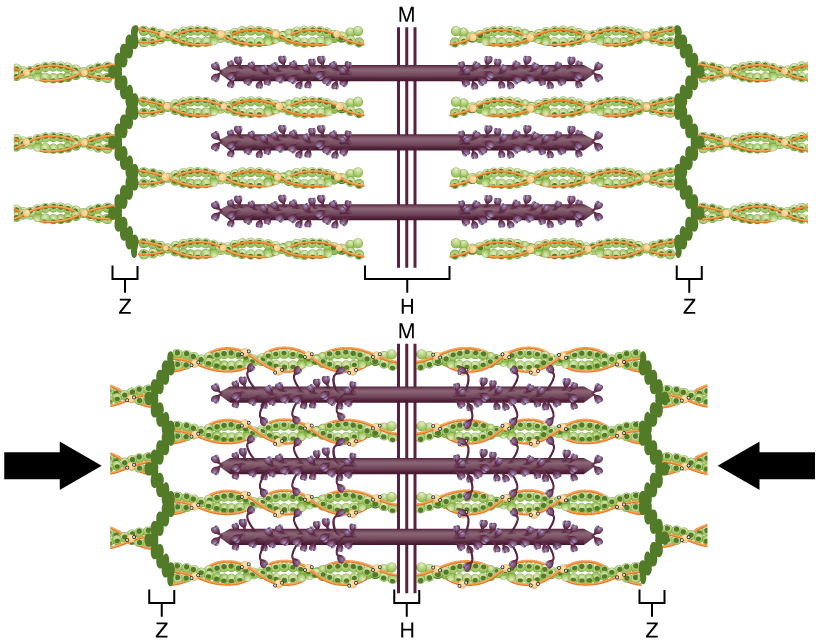
Crossbridge Cycling
Crossbridge cycling is a sequence of molecular events that underlies the sliding filament theory. There are many projections from the thick myosin filaments, each of which consists of two myosin heads (you can see the projections and heads in Figures 12.4.3 and 12.4.4). Each myosin head has binding sites for ATP (or the products of ATP hydrolysis: ADP and Pi) and for actin. The thin actin filaments also have binding sites for the myosin heads. A crossbridge forms when a myosin head binds with an actin filament.
The process of crossbridge cycling is shown in the video “Muscle Contraction 3D” by 3DBiology (below), and in Figure 12.4.4. A crossbridge cycle begins when the myosin head binds to an actin filament. ADP and Pi are also bound to the myosin head at this stage. Next, a power stroke moves the actin filament inward toward the center of sarcomere, thereby shortening the sarcomere. At the end of the power stroke, ADP and Pi are released from the myosin head, leaving the myosin head attached just to the thin filament until another ATP binds to the myosin head. When ATP binds to the myosin head, it causes the myosin head to detach from the actin. ATP is once again split into ADP and Pi and the energy released is used to move the myosin head into a “cocked” position. Once in this position, the myosin head can bind to the actin filament again, and another crossbridge cycle begins.
Muscle Contraction 3D, 3DBiology, 2017.
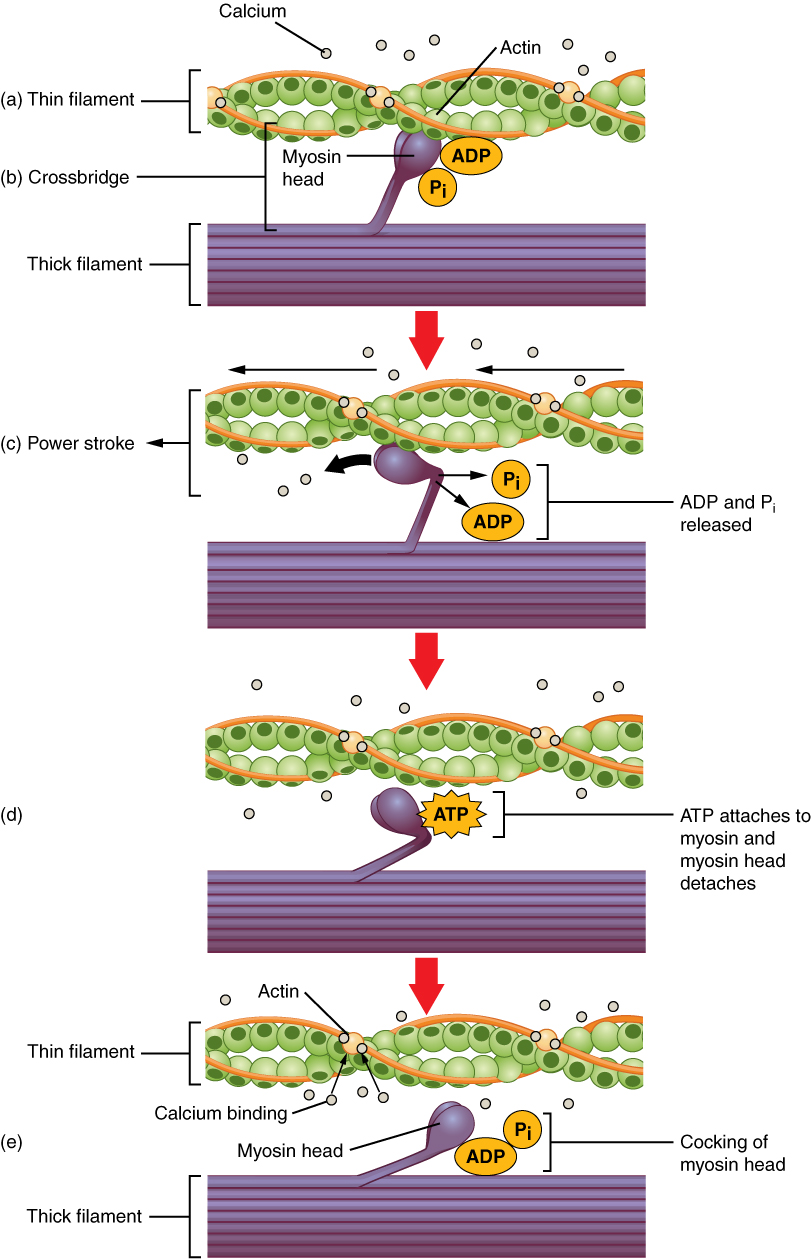
Energy for Muscle Contraction
According to the sliding filament theory, ATP is needed to provide the energy for a muscle contraction. Where does this ATP come from? Actually, there are multiple potential sources, as illustrated in Figure 12.4.5 below.
- As you can see from the first diagram, some ATP is already available in a resting muscle. As a muscle contraction starts, this ATP is used up in just a few seconds. More ATP is generated from creatine phosphate, but this ATP is used up rapidly as well. It’s gone in another 15 seconds or so.
- Glucose from the blood and glycogen stored in muscle can then be used to make more ATP. Glycogen breaks down to form glucose, and each glucose molecule produces two molecules of ATP and two molecules of pyruvate. Pyruvate (as pyruvic acid) can be used in aerobic respiration if oxygen is available. Alternatively, pyruvate can be used in anaerobic respiration, if oxygen is not available. The latter produces lactic acid, which may contribute to muscle fatigue. Anaerobic respiration typically occurs only during strenuous exercise when so much ATP is needed that sufficient oxygen cannot be delivered to the muscle to keep up.
- Resting or moderately active muscles can get most of the ATP they need for contractions by aerobic respiration. This process takes place in the mitochondria of muscle cells. In the process, glucose and oxygen react to produce carbon dioxide, water, and many molecules of ATP.
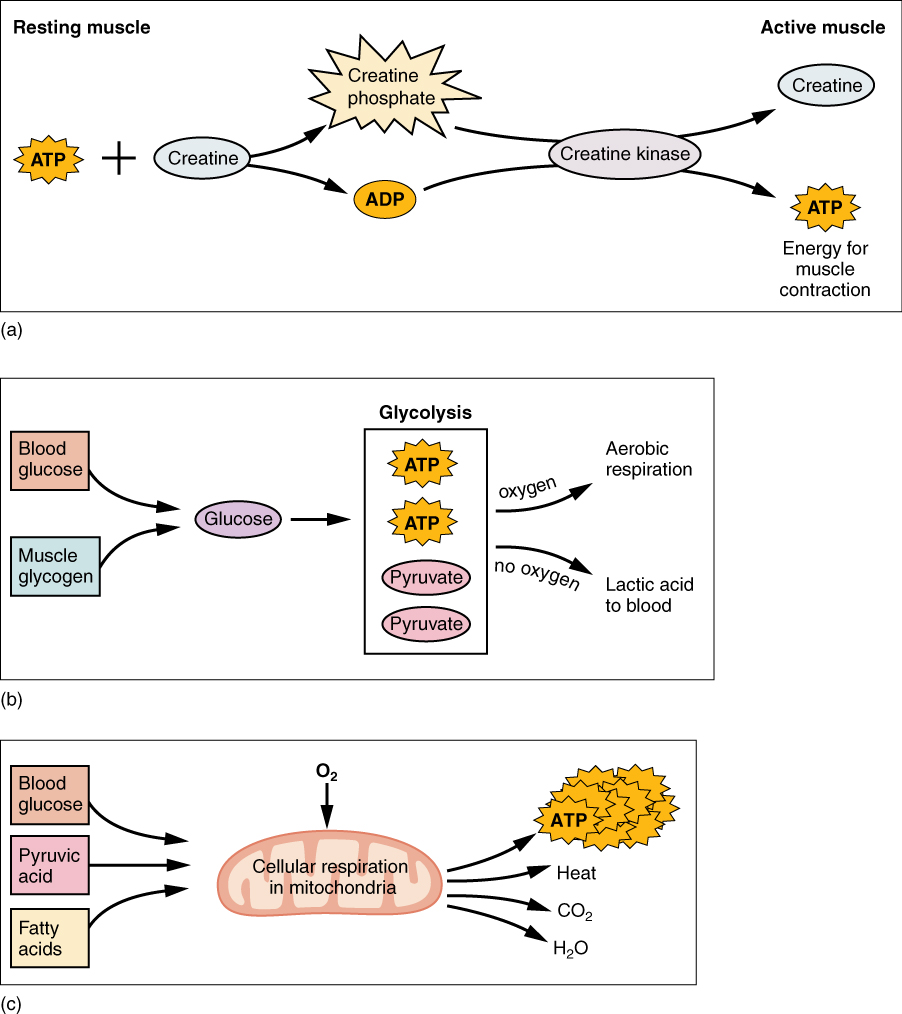
Feature: Human Biology in the News
Basic research on muscle contraction, especially if it is interesting and hopeful, is often in the news, because muscle contractions are involved in so many different body processes and disorders, including heart failure and stroke.
- Heart failure is a chronic condition in which cardiac muscle cells cannot contract forcefully enough to keep body cells adequately supplied with oxygen. According to a 2016 report by the Heart and Stroke Foundation of Canada, 600,000 Canadians are living with heart failure and each year, 50,000 new cases are diagnosed. Heart failure costs the Canadian medical system more than $2.8 billion annually. In 2016, researchers at the University of Texas Southwestern Medical Center identified a potential new target for the development of drugs to increase the strength of cardiac muscle contractions in patients with heart failure. The UT researchers found a previously unidentified protein involved in muscle contraction. The protein, which is very small, turns off the “brake” on the heart so it pumps blood more vigorously. At the molecular level, the protein affects the calcium-ion pump that controls muscle contraction. The scientists also found the same protein in slow-twitch skeletal muscle fibres. Interestingly, the protein is encoded by a stretch of mRNA that had been dismissed by scientists as non-coding RNA, commonly referred to as “junk” RNA. According to one of the researchers, “We dipped into the RNA ‘junk’ pile and came up with a hidden treasure.” This result is likely to lead to searches for additional treasures that might be hiding in the RNA junk pile.
- A stroke occurs when a blood clot lodges in an artery in the brain and cuts off blood flow to part of the brain. Approximately 6% of deaths in Canada are due to stroke and while men and women experiences strokes almost equally, women are more likely to die from a stroke. Damage from the clot associated with strokes would be reduced if the smooth muscles lining brain arteries relaxed following a stroke, because the arteries would dilate and allow greater blood flow to the brain. In a recent study undertaken at the Yale University School of Medicine, researchers determined that the muscles lining blood vessels in the brain actually contract after a stroke. This constricts the vessels, reduces blood flow to the brain, and appears to contribute to permanent brain damage. The hopeful takeaway of this finding is that it suggests a new target for stroke therapy.
12.4 Summary
- A muscle contraction is an increase in the tension or a decrease in the length of a muscle. A muscle contraction is isometric if muscle tension changes, but muscle length remains the same. It is isotonic if muscle length changes, but muscle tension remains the same.
- A skeletal muscle contraction begins with electrochemical stimulation of a muscle fibre by a motor neuron. This occurs at a chemical synapse called a neuromuscular junction. The neurotransmitter acetylcholine diffuses across the synaptic cleft and binds to receptors on the muscle fibre. This initiates a muscle contraction.
- Once stimulated, the protein filaments within the skeletal muscle fibre slide past each other to produce a contraction. The sliding filament theory is the most widely accepted explanation for how this occurs. According to this theory, thick myosin filaments repeatedly attach to and pull on thin actin filaments, thus shortening sarcomeres.
- Crossbridge cycling is a cycle of molecular events that underlies the sliding filament theory. Using energy in ATP, myosin heads repeatedly bind with and pull on actin filaments. This moves the actin filaments toward the center of a sarcomere, shortening the sarcomere and causing a muscle contraction.
- The ATP needed for a muscle contraction comes first from ATP already available in the cell, and more is generated from creatine phosphate. These sources are quickly used up. Glucose and glycogen can be broken down to form ATP and pyruvate. Pyruvate can then be used to produce ATP in aerobic respiration if oxygen is available, or it can be used in anaerobic respiration if oxygen is not available.
12.4 Review Questions
- What is a skeletal muscle contraction?
-
- Explain sliding filament theory and describe crossbridge cycling.
- If the acetylcholine receptors on muscle fibres were blocked by a drug, what do you think this would do to muscle contraction? Explain your answer.
- Explain how crossbridge cycling and sliding filament theory are related to each other.
- When does anaerobic respiration typically occur in human muscle cells?
- If there were no ATP available in a muscle, how would this affect crossbridge cycling? What would this do to muscle contraction?
12.4 Explore More
The Mechanism of Muscle Contraction: Sarcomeres, Action Potential, and the Neuromuscular Junction, Professor Dave Explains, 2019.
Aerobic vs Anaerobic Difference, Dorian Wilson, 2017.
Attributions
Figure 12.4.1
Armwrestling_Championships by Jnadler1 on Wikimedia Commons is used under a CC BY-SA 3.0 (https://creativecommons.org/licenses/by-sa/3.0) license.
Figure 12.4.2
Motor_End_Plate_and_Innervation by OpenStax on Wikimedia Commons is used under a CC BY 4.0 (https://creativecommons.org/licenses/by 4.0) license.
Figure 12.4.3
Sliding_Filament_Model_of_Muscle_Contraction by OpenStax on Wikimedia Commons is used under a CC BY 4.0 (https://creativecommons.org/licenses/by 4.0) license.
Figure 12.4.4
Skeletal_Muscle_Contraction by OpenStax on Wikimedia Commons is used under a CC BY 4.0 (https://creativecommons.org/licenses/by 4.0) license.
Figure 12.4.5
Muscle_Metabolism by OpenStax on Wikimedia Commons is used under a CC BY 4.0 (https://creativecommons.org/licenses/by 4.0) license.
References
3DBiology. (2017). Muscle contraction 3D. YouTube. https://www.youtube.com/watch?v=GrHsiHazpsw
Betts, J. G., Young, K.A., Wise, J.A., Johnson, E., Poe, B., Kruse, D.H., Korol, O., Johnson, J.E., Womble, M., DeSaix, P. (2016, May 27). Figure 10.6 Motor end-plate and innervation [digital image]. In Anatomy and Physiology (Section 10.2). OpenStax. https://openstax.org/books/anatomy-and-physiology/pages/10-2-skeletal-muscle
Betts, J. G., Young, K.A., Wise, J.A., Johnson, E., Poe, B., Kruse, D.H., Korol, O., Johnson, J.E., Womble, M., DeSaix, P. (2016, May 27). Figure 10.10 The sliding filament model of muscle contraction [digital image]. In Anatomy and Physiology (Section 10.3). OpenStax. https://openstax.org/books/anatomy-and-physiology/pages/10-3-muscle-fiber-contraction-and-relaxation
Betts, J. G., Young, K.A., Wise, J.A., Johnson, E., Poe, B., Kruse, D.H., Korol, O., Johnson, J.E., Womble, M., DeSaix, P. (2016, May 27). Figure 10.11 Skeletal muscle contraction [digital image]. In Anatomy and Physiology (Section 10.3). OpenStax. https://openstax.org/books/anatomy-and-physiology/pages/10-3-muscle-fiber-contraction-and-relaxation
Betts, J. G., Young, K.A., Wise, J.A., Johnson, E., Poe, B., Kruse, D.H., Korol, O., Johnson, J.E., Womble, M., DeSaix, P. (2016, May 27). Figure 10.12 Muscle metabolism [digital image]. In Anatomy and Physiology (Section 10.3). OpenStax. https://openstax.org/books/anatomy-and-physiology/pages/10-3-muscle-fiber-contraction-and-relaxation
Dorian Wilson. (2017, March 8). Aerobic vs anaerobic difference. YouTube. https://www.youtube.com/watch?v=8Y_FdjI2v4I&feature=youtu.be
Heart and Stroke Foundation. (2016). 2016 Report on the health of Canadians: The burden of heart failure. https://www.heartandstroke.ca/-/media/pdf-files/canada/2017-heart-month/heartandstroke-reportonhealth-2016.ashx?la=en
Hill, R. A., Tong, L., Yuan, P., Murikinati, S., Gupta, S., & Grutzendler, J. (2015). Regional blood flow in the normal and ischemic brain is controlled by arteriolar smooth muscle cell contractility and not by capillary pericytes. Neuron, 87(1), 95–110. https://doi.org/10.1016/j.neuron.2015.06.001
UTSouthwestern Newsroom. (2016, January 14). Researchers find a small protein that plays a big role in heart muscle contraction [online article]. https://www.utsouthwestern.edu/newsroom/articles/year-2016/dworf-protein-olson.html
What we do. (n.d.). Heart and Stroke Foundation of Canada. https://www.heartandstroke.ca/what-we-do
Increase in the tension or decrease in the length of a muscle that occurs when muscle fibers receive stimulation from the nervous system.
Referring to a muscle contraction in which muscle tension increases but muscle length remains the same.
Referring to a muscle contraction in which muscle length decreases but muscle tension remains the same.
A division of the peripheral nervous system that controls voluntary activities.
A type of neuron that carries nerve impulses from the central nervous system to muscles and glands; also called efferent neuron.
A chemical synapse where a motor neuron transmits a signal to a muscle fiber to initiate a muscle contraction.
Reversal of electrical charge across the membrane of a resting neuron that travels down the axon of the neuron as a nerve impulse.
An organic chemical that functions in the brain and body of many types of animals (and humans) as a neurotransmitter—a chemical message released by nerve cells to send signals to other cells, such as neurons, muscle cells and gland cells.
A space that separates two neurons. It forms a junction between two or more neurons and helps nerve impulse pass from one neuron to the other.
A protein on a cell membrane or inside of a cell that binds with a hormone, neurotransmitter, or other chemical signal to produce a response.
A protein that forms (together with myosin) the contractile filaments of muscle cells, and is also involved in motion in other types of cells.
A fibrous protein that forms (together with actin) the contractile filaments of muscle cells and is also involved in motion in other types of cells.
A theory that explains muscle contraction by the sliding of myosin filaments over actin filaments within muscle fibers.
The basic functional unit of skeletal and cardiac muscles, containing actin and myosin protein filaments that slide over one another to produce a shortening of the sarcomere resulting in a muscle contraction.
A sequence of molecular events that forms crossbridges between myosin and actin filaments in muscle fibers, allowing for muscle contraction. "Heads" on the myosin filaments essentially form a connection with specific locations on the actin, and then the head bends in order to pull the myosin strand along the actin to shorten the sarcomere.
A complex organic chemical that provides energy to drive many processes in living cells, e.g. muscle contraction, nerve impulse propagation, and chemical synthesis. Found in all forms of life, ATP is often referred to as the "molecular unit of currency" of intracellular energy transfer.
An organic compound of creatine and phosphate, also known as phosphocreatine, which when hydrolyzed (split apart) releases energy for muscle contraction.
Glucose (also called dextrose) is a simple sugar with the molecular formula C6H12O6. Glucose is the most abundant monosaccharide, a subcategory of carbohydrates. Glucose is mainly made by plants and most algae during photosynthesis from water and carbon dioxide, using energy from sunlight.
A multi-branched polysaccharide of glucose that serves as a form of energy storage in animals, fungi, and bacteria.
The process of producing cellular energy involving oxygen. Cells break down food in the mitochondria in a long, multi-step process that produces roughly 36 ATP. The first step in is glycolysis, the second is the Krebs cycle and the third is the electron transport system.
Respiration using electron acceptors other than molecular oxygen. Although oxygen is not the final electron acceptor, the process still uses a respiratory electron transport chain.
A double-membrane-bound organelle found in most eukaryotic organisms. Mitochondria convert oxygen and nutrients into adenosine triphosphate (ATP). ATP is the chemical energy "currency" of the cell that powers the cell's metabolic activities.
A term used to describe a heart that cannot keep up with its workload. The body may not get the oxygen it needs. Heart failure is a serious condition, and usually there's no cure.
A cerebrovascular accident in which a broken artery or blood clot results in lack of blood flow to part of the brain, causing death of brain cells.
A type of chemical that transmits signals from the axon of a neuron to another cell across a synapse.